Delivery of Reactive Oxygen Species (ROS)
Controlled H₂O₂ Generation Using BRICK Systems
Reactive oxygen species (ROS) such as hydrogen peroxide (H₂O₂) play a central role in cell signaling, oxidative stress, and immune responses. In many biological contexts, ROS function not only as damaging agents but also as key regulators of both physiological and pathological processes.
Delivering ROS in vitro is technically challenging. Conventional methods — such as pipetting hydrogen peroxide into the system — lead to a sharp, non-physiological concentration spike, followed by rapid degradation due to enzymatic activity and reactions with biomolecules. This poorly mimics the spatial and temporal dynamics of ROS observed in living tissues.
Our solution: by using the BRICK and BRICKmicro platforms, equipped with PEDOT:PSS working electrodes and carefully tuned electrochemical protocols, we achieve selective electrochemical reduction of dissolved oxygen to hydrogen peroxide. This enables precise, tunable, and sustained delivery of H₂O₂ into biological systems — opening new possibilities for physiologically relevant ROS studies.
Electrochemical H₂O₂ Delivery in Microplates
BRICK System
The Double Well BRICK system allows for peroxide generation in standard 24-, 48-, or 96-well microplates. Each of the 8 channels can:
Apply the same protocol across wells for statistically robust replicates
Or apply individual protocols to screen different peroxide delivery profiles
The Double Well configuration separates cathodic and anodic reactions using a salt bridge, effectively:
Preventing peroxide oxidation at the counter electrode
Isolating oxidative side reactions
This makes BRICK a powerful platform for high-throughput ROS studies in a familiar cell culture format.
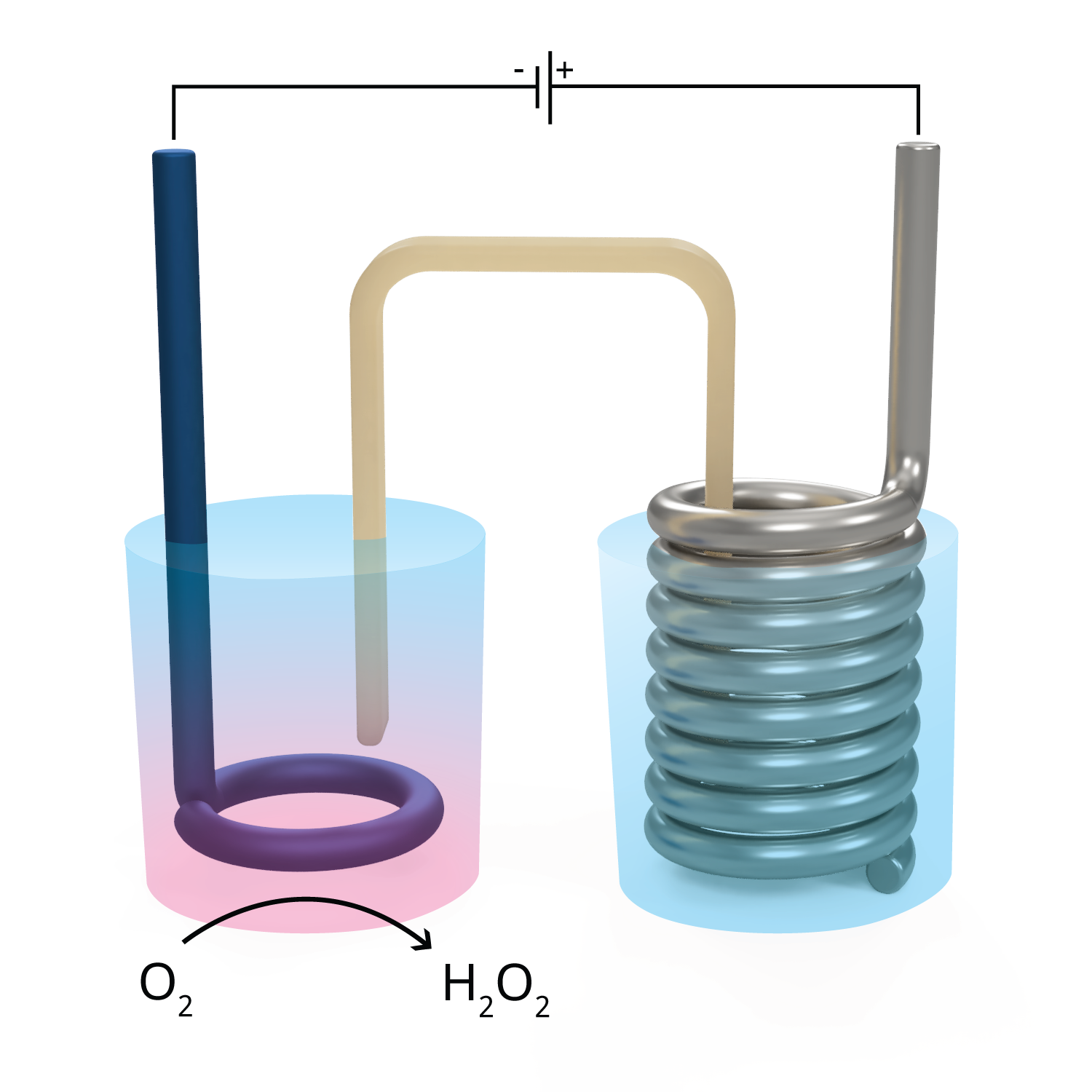
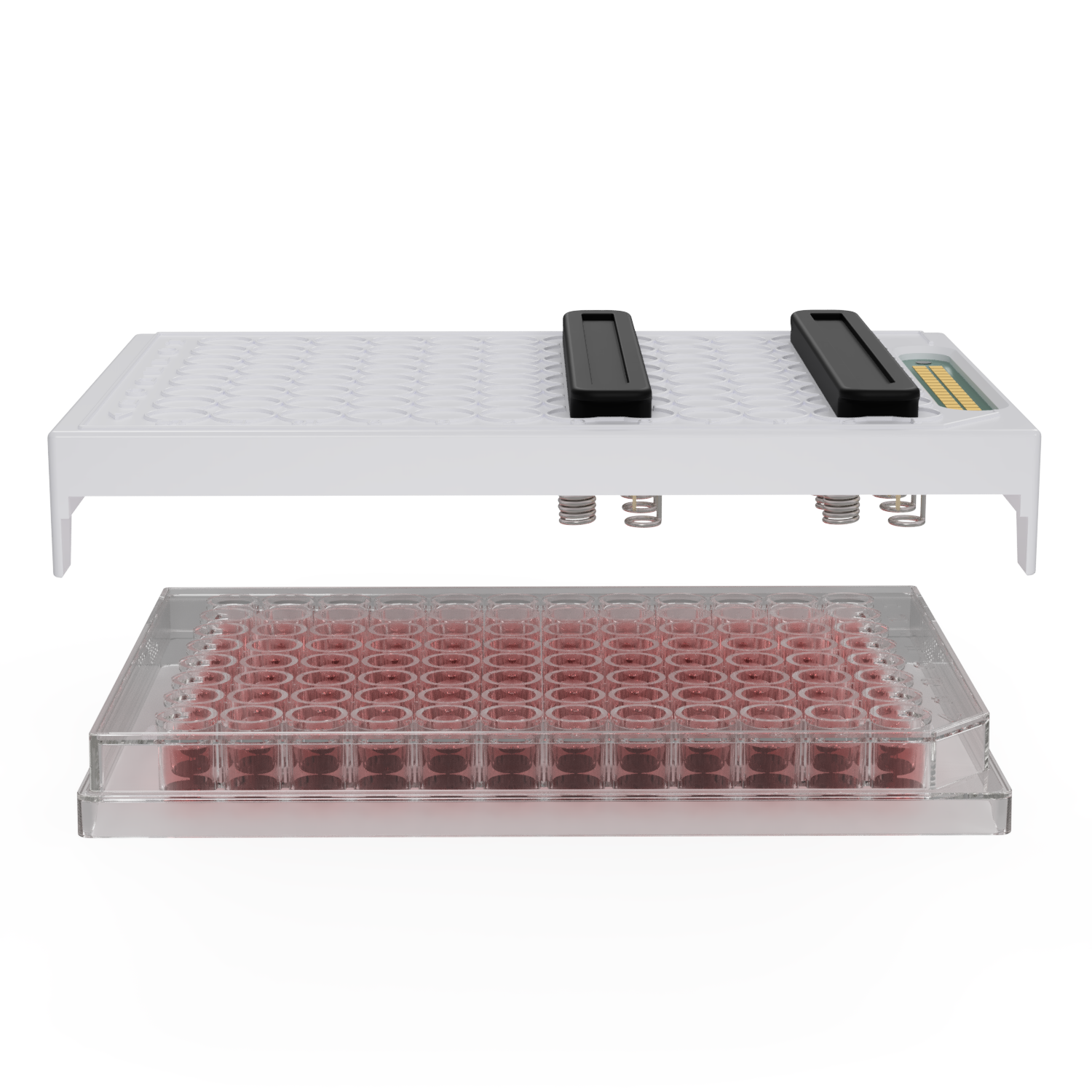
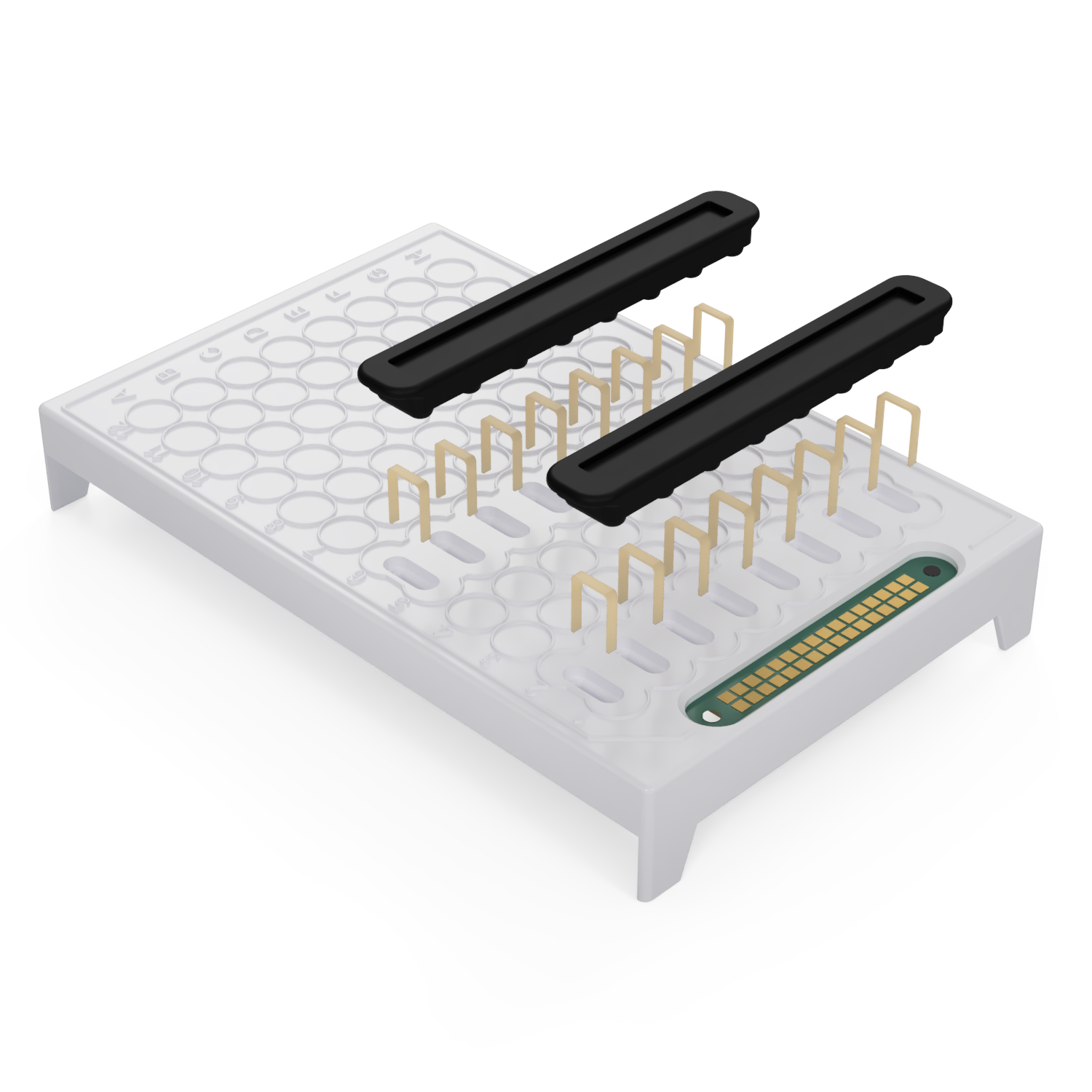
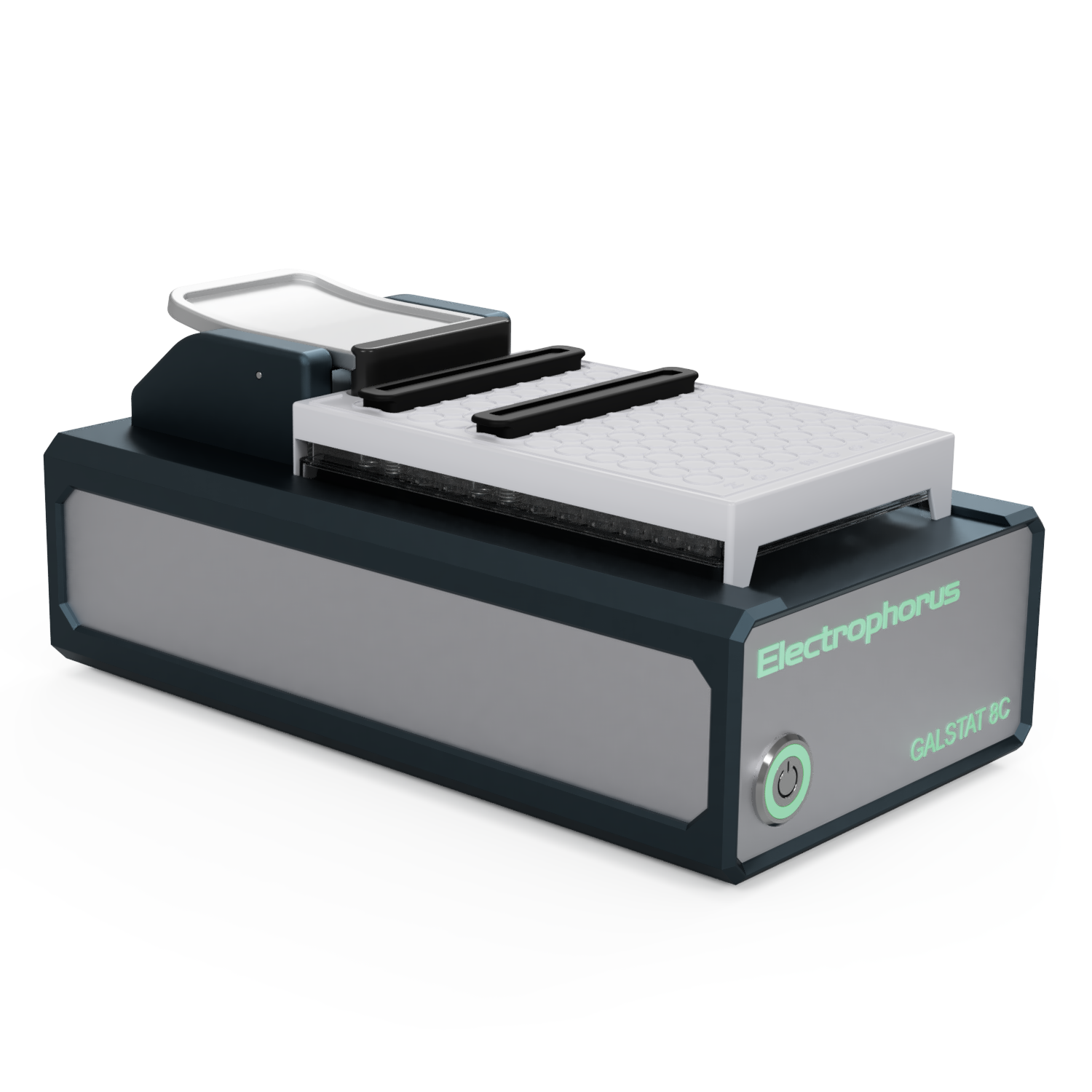
ROS Gradient Formation for Live-Cell Imaging
BRICK micro System
The BRICKmicro system allows for the formation of controlled H₂O₂ gradients on microscope slides, enabling direct observation of redox effects at the cellular level.
By customizing electrode geometry (e.g., parallel or concentric designs) and applying appropriate protocols, you can:
Generate gradients between cathodic peroxide-producing and anodic peroxide-consuming electrodes
Study how cells respond to ROS gradients in real time under the microscope
Use all 8 channels to run parallel replicates or screen multiple gradient profiles
This opens exciting possibilities in studies of cell migration, signaling thresholds, oxidative stress responses, and more.
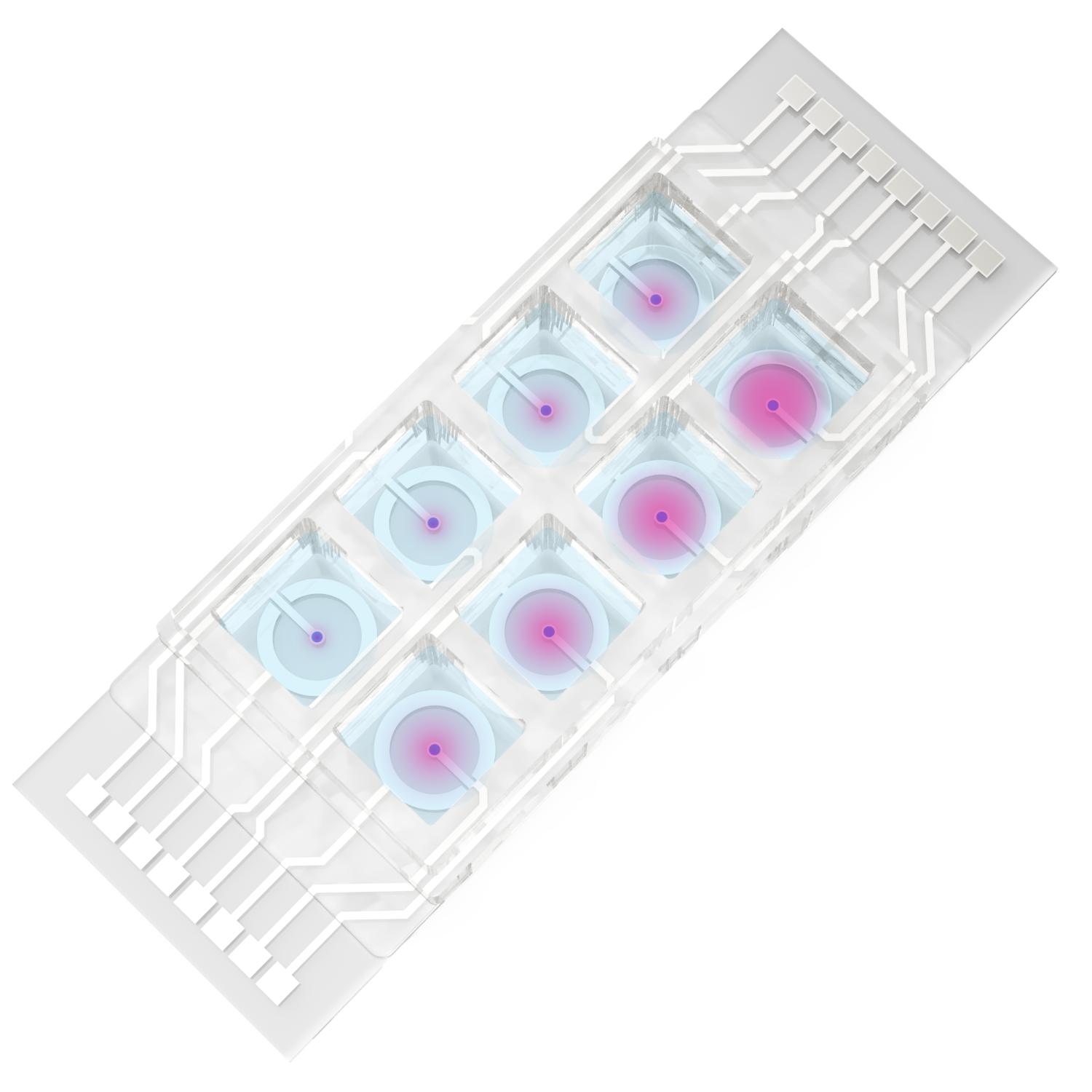
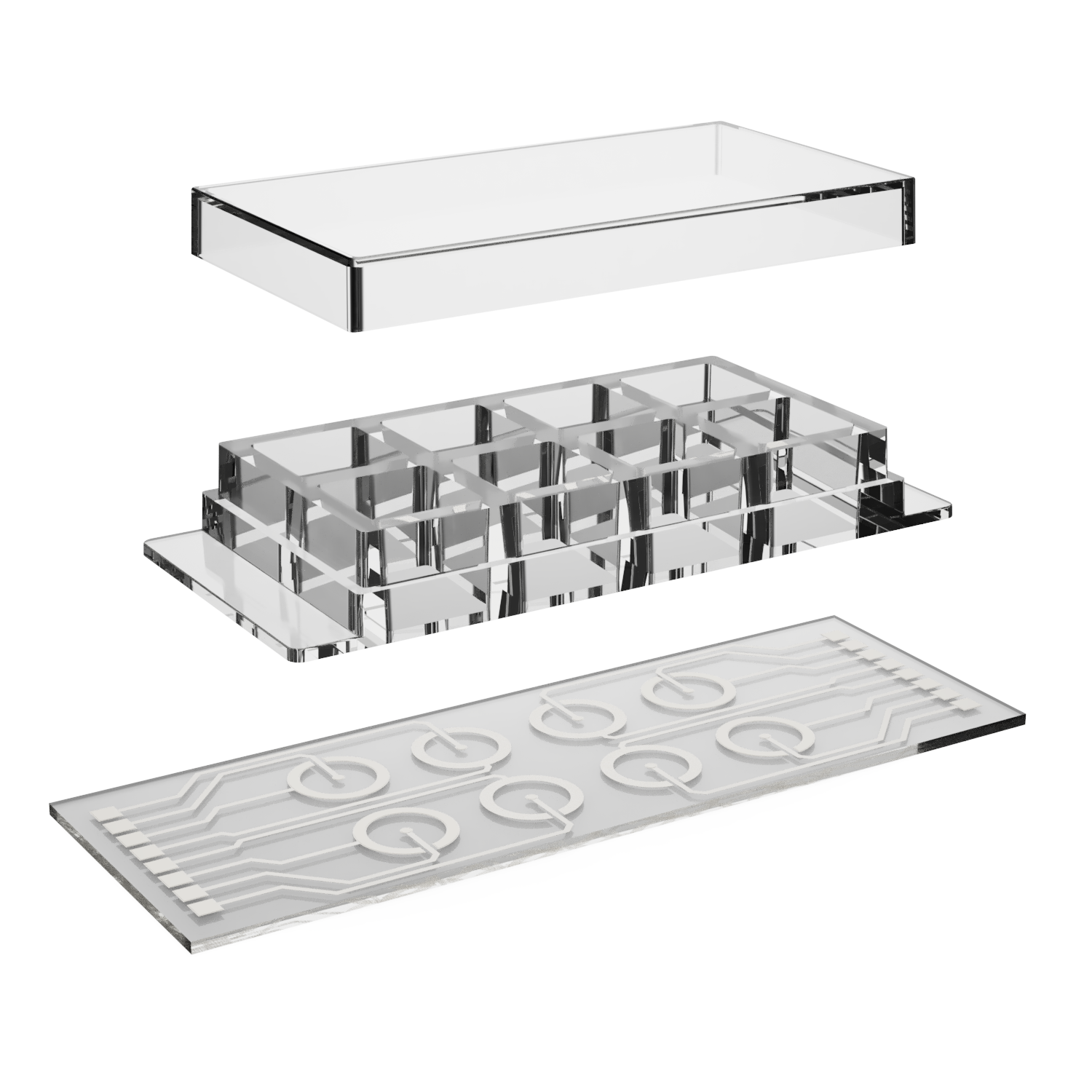
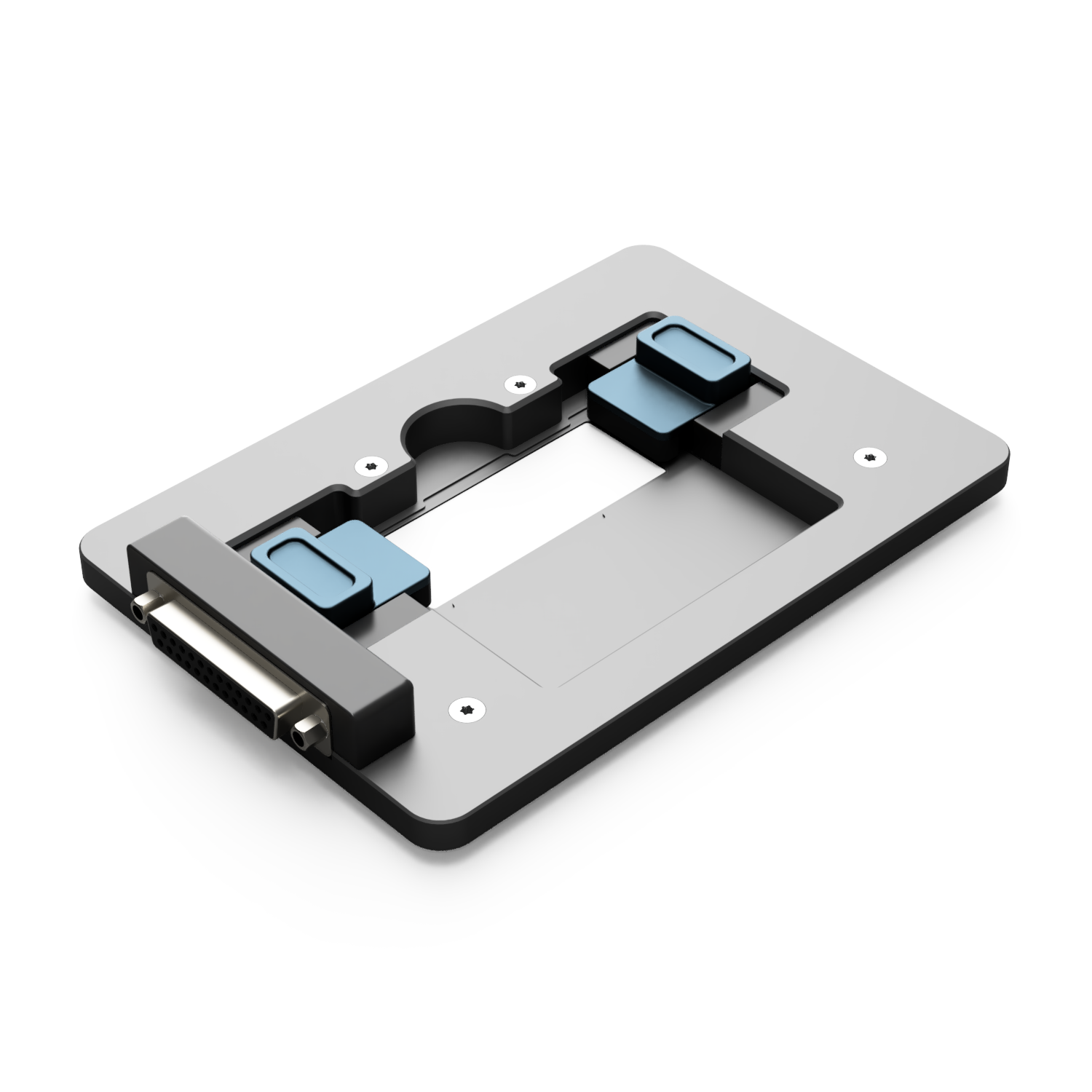
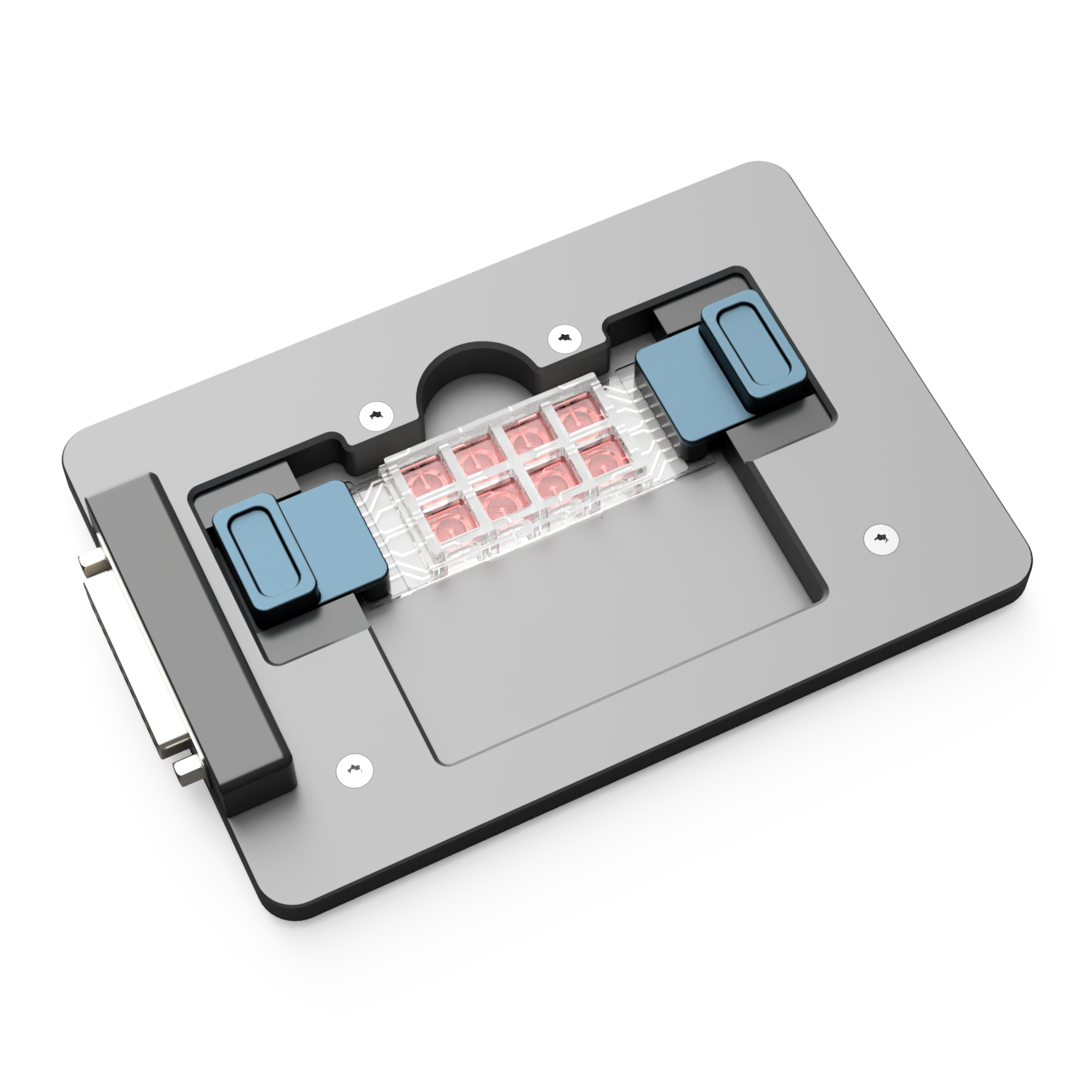
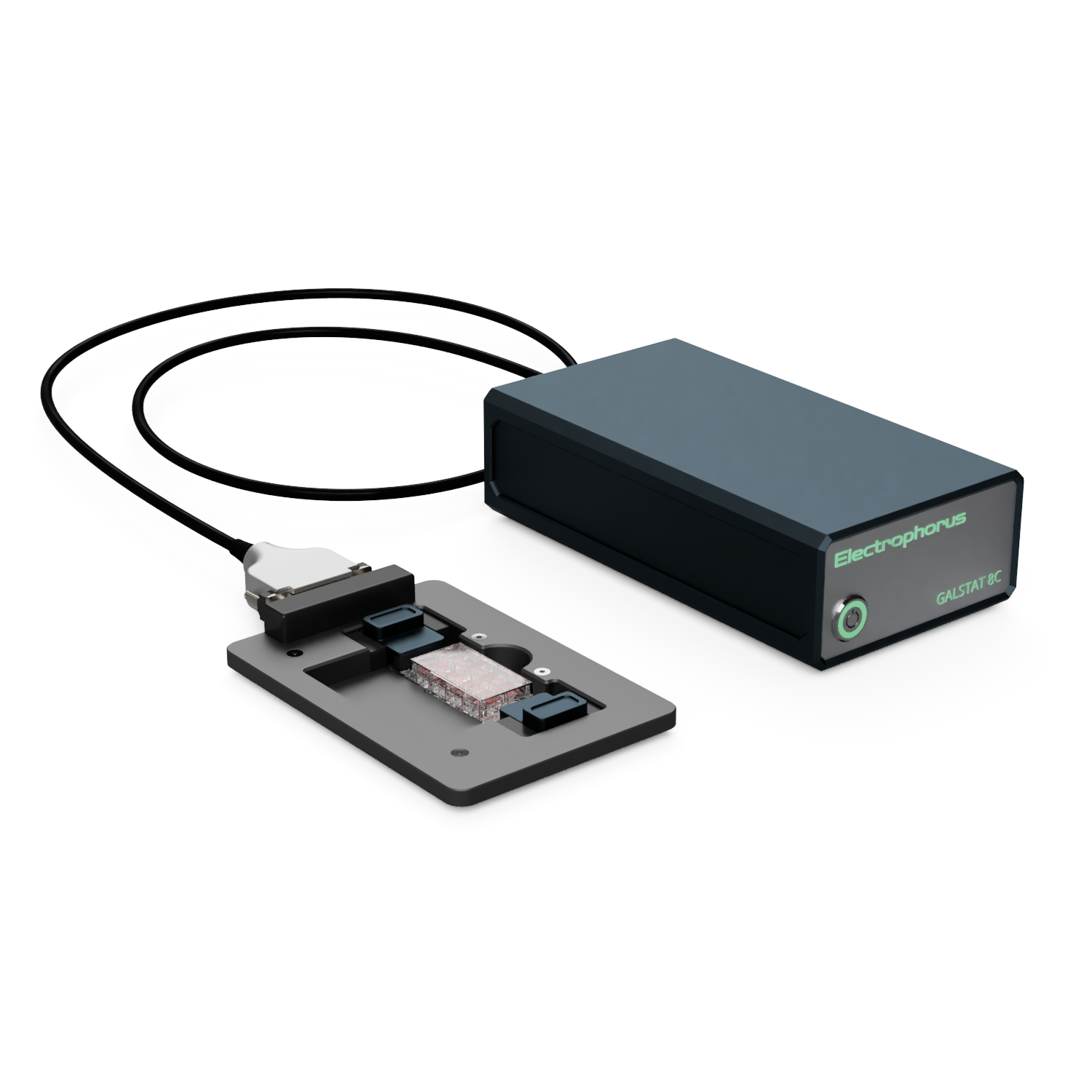
How It Works
At the core of our method is the oxygen reduction reaction (ORR) on PEDOT:PSS electrodes, which selectively catalyzes the following half-reaction [1]:
O₂ + 2 H⁺ + 2 e⁻ → H₂O₂
This reaction, widely used in industry [2], remains underutilized in biology [3] [4] [5]— despite its high selectivity, low energy requirements, and absence of harmful byproducts.
The resulting peroxide concentration is governed by:
System architecture (e.g., electrode geometry and well size)
Electrochemical protocol (applied potential/current and duration)
Oxygen availability (oxygen consumed is naturally replenished by passive diffusion)
By operating well below the electrolysis threshold, this method avoids pH shifts, gas formation, and toxic side products, making it highly compatible with sensitive biological systems.
What Makes Our System Unique
Unlike injecting peroxide directly — which leads to transient exposure and exponential decay — our electrochemical approach enables:
Steady-state peroxide levels maintained over extended periods
Fine-tuned control over concentration, from a few µM to several hundred µM
Programmable spatio-temporal profiles, including concentration gradients
Sustained peroxide delivery — limited only by oxygen diffusion and system architecture
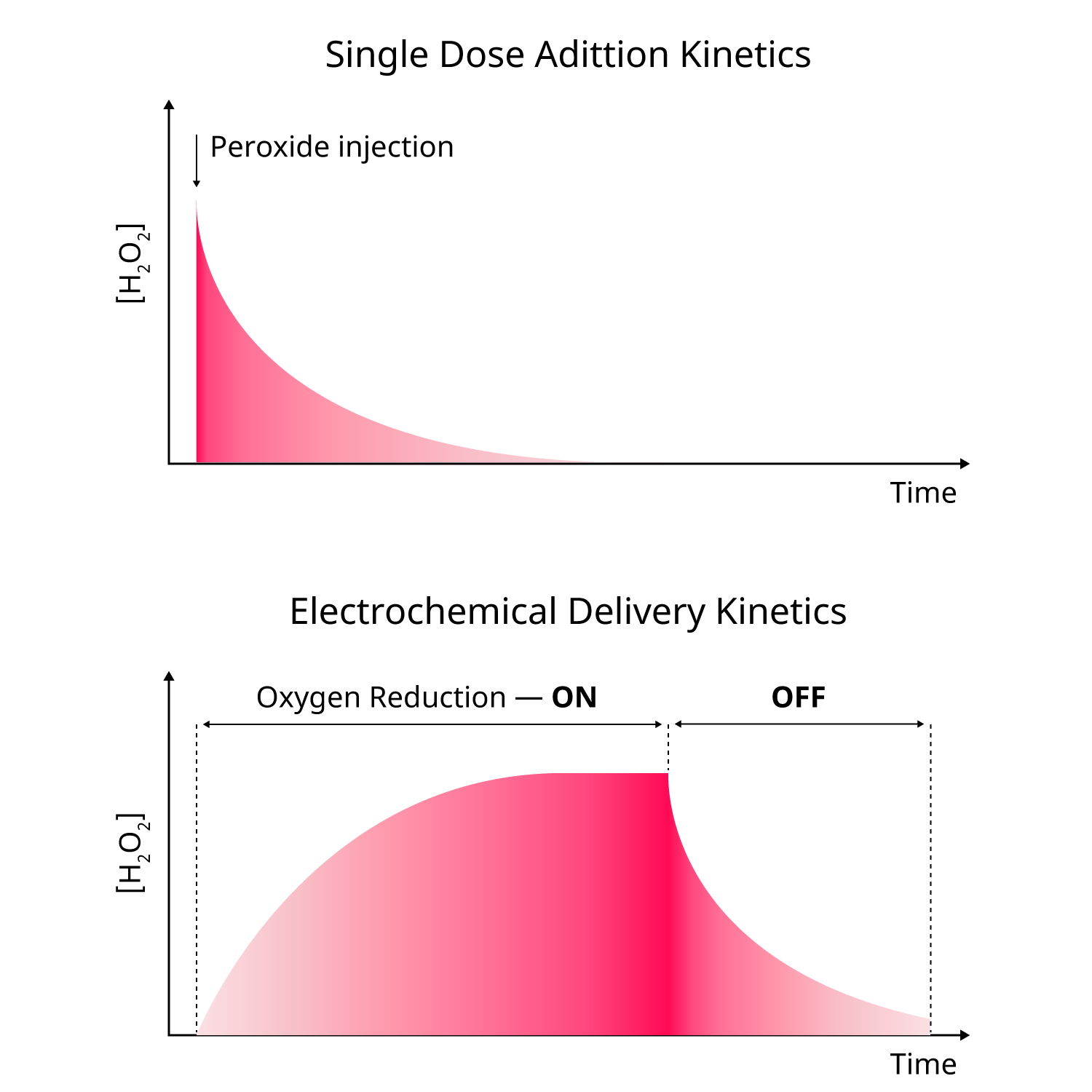
Get Started with Electrochemical ROS Delivery
Interested in using electrochemically controlled ROS delivery in your research?
Book a call with us to explore how BRICK or BRICK micro can help you design reproducible, physiologically relevant redox experiments.
References
[1] Ehlich J, Migliaccio L, Sahalianov I, Nikić M, Brodský J, Gablech I, Vu XT, Ingebrandt S, Głowacki ED. Direct measurement of oxygen reduction reactions at neurostimulation electrodes. J Neural Eng. 2022 Jun 27;19(3). doi: 10.1088/1741-2552/ac77c0. PMID: 35688124.
[2] Perry, S.C., Pangotra, D., Vieira, L. et al. Electrochemical synthesis of hydrogen peroxide from water and oxygen. Nat Rev Chem 3, 442–458 (2019). https://doi.org/10.1038/s41570-019-0110-6
[3] Istanbullu O, Babauta J, Duc Nguyen H, Beyenal H. Electrochemical biofilm control: mechanism of action. Biofouling. 2012;28(8):769-78. doi: 10.1080/08927014.2012.707651. PMID: 22827804; PMCID: PMC4247835.
[4] Sultana ST, Atci E, Babauta JT, Falghoush AM, Snekvik KR, Call DR, Beyenal H. Electrochemical scaffold generates localized, low concentration of hydrogen peroxide that inhibits bacterial pathogens and biofilms. Sci Rep. 2015 Oct 14;5:14908. doi: 10.1038/srep14908. PMID: 26464174; PMCID: PMC4604468.
[5] Raval YS, Fleming D, Mohamed A, Karau MJ, Mandrekar JN, Schuetz AN, Greenwood Quaintance KE, Beyenal H, Patel R. In Vivo Activity of Hydrogen-Peroxide Generating Electrochemical Bandage Against Murine Wound Infections. Adv Ther (Weinh). 2023 May;6(5):2300059. doi: 10.1002/adtp.202300059. Epub 2023 Mar 3. PMID: 37485434; PMCID: PMC10361686.